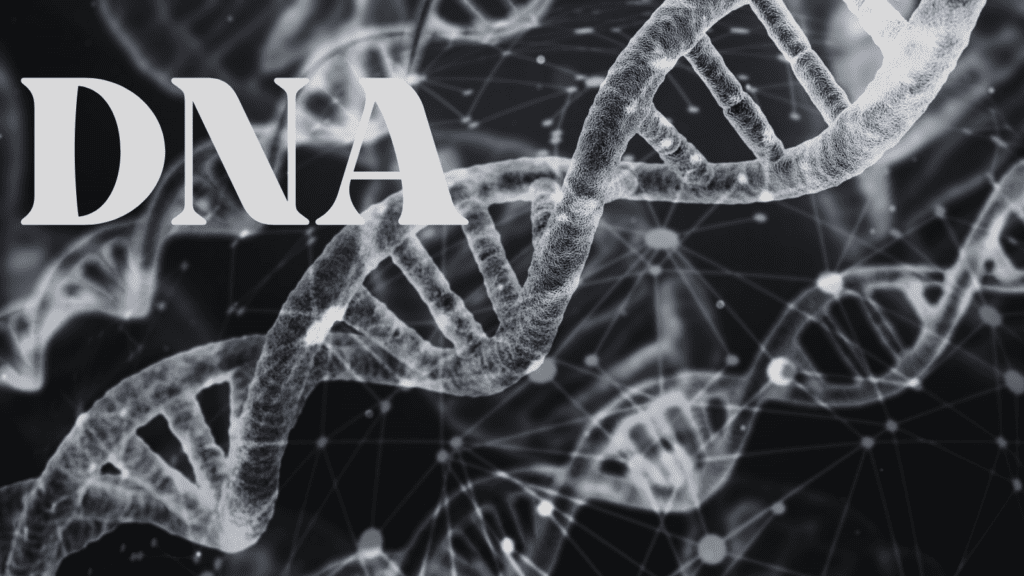
What is DNA Or Deoxyribonucleic Acid?
DNA, or Deoxyribonucleic Acid, is a fundamental molecule that serves as the hereditary material in all living organisms, ranging from the simplest bacteria to complex multicellular organisms. The structure of DNA is a double helix, resembling a twisted ladder, which was famously elucidated by James Watson and Francis Crick in 1953. This unique conformation plays a critical role in both the functionality and stability of the genetic material.
The basic components of DNA are nucleotides, which are the building blocks of this crucial molecule. Each nucleotide consists of three essential parts: a phosphate group, a deoxyribose sugar, and a nitrogenous base. There are four different types of nitrogenous bases found in DNA: adenine (A), thymine (T), cytosine (C), and guanine (G). The sequence of these bases encodes the genetic information necessary for the development, functioning, and reproduction of all living organisms.
The two strands of DNA are held together by hydrogen bonds between complementary nitrogenous bases, specifically adenine pairs with thymine, and cytosine pairs with guanine. This pairing is vital for the accurate replication of DNA during cell division, ensuring that genetic information is faithfully passed from one generation to the next.
Beyond its role in heredity, DNA also serves as a template for the synthesis of RNA, which subsequently guides the production of proteins, the workhorses of the cell. This central dogma of molecular biology underscores the importance of DNA not only as a repository of genetic information but also as a blueprint for the biological processes that sustain life.
Understanding the structure and function of DNA provides insights into the complexities of living organisms and has immense implications in fields such as genetics, biotechnology, and medicine.
The Structure of DNA
The structural integrity of DNA, or deoxyribonucleic acid, is foundational to its role as the molecule of life. At its core, DNA is composed of nucleotides, which are the building blocks that facilitate the storage and transmission of genetic information. Each nucleotide consists of three main components: a phosphate group, a five-carbon sugar known as deoxyribose, and a nitrogenous base. There are four distinct nitrogenous bases in DNA: adenine (A), thymine (T), cytosine (C), and guanine (G). These bases play a pivotal role in encoding the genetic instructions required for the growth, development, and functioning of living organisms.
The arrangement of these nucleotide bases is not random; rather, they pair in specific ways—adenine pairs with thymine, while cytosine pairs with guanine. This base pairing is essential for the double-helical structure of DNA, facilitating the formation of complementary strands. The precise alignment and attraction between these bases, known as hydrogen bonding, ensure that the genetic code is accurately replicated during cell division and maintained throughout generations. This phenomenon underscores the importance of base pairing in both genetic fidelity and the overall stability of the DNA molecule.
Moreover, DNA strands are oriented in opposite directions, a configuration referred to as antiparallelism. This structural trait is significant because it allows enzymes involved in DNA replication to synthesize new strands efficiently. The antiparallel nature of the strands also has implications for post-replication processes, including transcription and translation, whereby the information from the DNA is utilized to produce proteins. The visualization of DNA through 3D models and diagrams has been instrumental in enhancing our comprehension of this intricate structure, making it evident that the molecular framework of DNA is not just a physical configuration but a vital aspect of biological function.
How DNA Replication Works
DNA replication is a crucial cellular process that ensures the accurate duplication of the genetic material before cell division. This process is characterized as semi-conservative, meaning that each new DNA molecule consists of one original strand and one newly synthesized strand. The replication process can be divided into three primary stages: initiation, elongation, and termination, each facilitated by specific enzymes and proteins.
The initiation phase begins with the unwinding of the double helix structure of DNA, a task primarily managed by the enzyme helicase. This enzyme separates the two strands of the DNA, creating a replication fork. Single-strand binding proteins then attach to the separated strands to prevent them from re-annealing. Once the strands are separated, the enzyme primase lays down a short RNA primer, which is essential for DNA polymerase to begin synthesizing new DNA strands. This primer provides a starting point that DNA polymerase requires to add nucleotides sequentially.
In the elongation phase, DNA polymerase plays a vital role by adding complementary nucleotides to the growing new strand, following the base-pairing rules where adenine pairs with thymine and cytosine pairs with guanine. This enzyme also possesses proofreading capabilities, enabling it to correct any errors that may arise during replication. Importantly, replication occurs in a 5’ to 3’ direction, meaning that one strand, called the leading strand, is synthesized continuously, while the other strand, known as the lagging strand, is synthesized in short fragments, later joined together by the enzyme ligase.
The termination phase occurs when the DNA polymerase reaches the end of a DNA strand or encounters a specific sequence that signals the completion of replication. At this stage, the RNA primers are replaced with DNA, and DNA ligase finalizes the process by sealing any gaps between the newly synthesized fragments. Thus, through this intricate mechanism involving multiple enzymes and steps, DNA replication ensures the faithful transmission of genetic information, laying the foundation for cellular function and heredity.
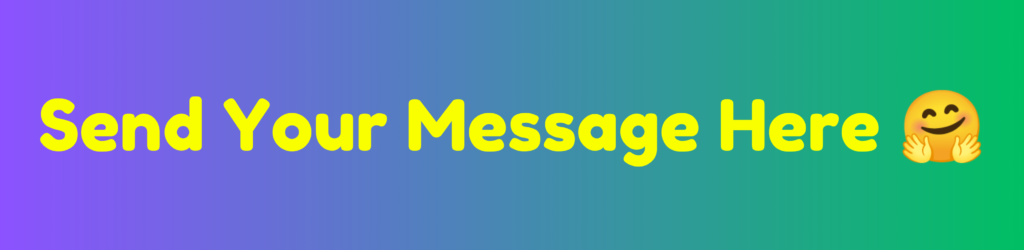
Genes and Their Role in Inheritance
Genes are fundamental units of heredity and play a crucial role in the inheritance of traits from one generation to the next. These segments of DNA are responsible for encoding the information necessary for the development, functioning, and reproduction of organisms. Each gene consists of a specific sequence of nucleotides, which ultimately translates into proteins that perform vital functions in the body.
The genetic makeup of an individual is referred to as the genotype, which can be expressed in various ways. The observable characteristics or traits resulting from the genotype are known as the phenotype. For instance, in humans, traits like eye color, hair type, and height are all influenced by an individual’s genetic composition. Understanding the distinction between genotype and phenotype is essential in genetics, particularly when analyzing how traits are passed on through generations.
According to Mendelian inheritance principles, traits are determined by alleles, which are different forms of a gene. Alleles can be classified as dominant or recessive. A dominant allele expresses its effect even if only one copy is present, while a recessive allele expresses its effect only when two copies are present (one from each parent). This can be illustrated using simple genetic diagrams such as Punnett squares, which help predict the probability of offspring inheriting specific traits based on parental genotypes.
For instance, if we consider a trait controlled by a single gene with two alleles, one dominant (A) and one recessive (a), the possible genotypes of the offspring can be visualized easily. If one parent has the genotype AA and the other has aa, all offspring will inherit the genotype Aa, manifesting the dominant phenotype. Such patterns of inheritance elucidate how genetic traits are transmitted and help in predicting variations within populations.
Mutations: The Changes in DNA
Mutations are alterations in the nucleotide sequence of DNA, and they can occur for various reasons, encompassing both external and internal influences. These changes can arise from environmental factors such as radiation, chemicals, or viruses, as well as from errors that may occur during DNA replication. Essentially, mutations can be classified into two primary categories: point mutations and chromosomal mutations. Point mutations involve a change in a single nucleotide, which can result in the alteration of a single amino acid in a protein. Such changes can have a range of effects, from benign to severe, depending on the role of that specific amino acid in the protein’s function.
On the other hand, chromosomal mutations encompass larger-scale alterations, including deletions, duplications, inversions, or translocations of entire sections of chromosomes. These mutations can lead to significant genetic variation, potentially resulting in phenotypic differences or even the development of diseases. For example, a deletion in chromosome 5 can lead to Cri du Chat syndrome, which is characterized by developmental delays and distinct physical features. Similarly, an inversion on chromosome 9 has been linked to an increased risk of certain cancers.
The impact of mutations on organisms is multifaceted. They can contribute to evolutionary processes by creating genetic diversity that can be acted upon by natural selection. However, many mutations are deleterious, resulting in genetic disorders that may be inherited or appear sporadically in populations. The study of mutations not only enhances our understanding of genetic variation but also informs medical research aimed at identifying and potentially rectifying the underlying causes of hereditary diseases. Through careful examination of mutation patterns, researchers can devise therapies that target specific genetic anomalies.
Applications of DNA Technology
The advancements in DNA technology have ushered in a new era of possibilities across various fields, significantly influencing modern science and healthcare. One of the most prominent applications is genetic engineering, which involves altering the genetic makeup of organisms to enhance or introduce desirable traits. This technology has found widespread use in agriculture, allowing for the development of genetically modified organisms (GMOs) that exhibit increased resilience to pests and environmental stressors, potentially leading to improved food security.
Another remarkable development is the CRISPR-Cas9 technique, which serves as a revolutionary tool for editing genes with precision. Originally discovered in bacteria as a defense mechanism against viruses, CRISPR enables scientists to target specific DNA sequences and make alterations swiftly and with high accuracy. This innovation holds immense potential for treating genetic disorders, advancing research in gene therapy, and even combating diseases such as cancer by editing the genes responsible for tumor growth.
Forensics is yet another critical area where DNA technology plays a crucial role. From crime scene investigations to paternity testing, DNA profiling has transformed how legal systems operate, offering compelling evidence that can lead to the identification of suspects or exoneration of the innocent. Forensic scientists analyze an individual’s unique DNA patterns, which serve as a reliable means of establishing identity, thus enhancing the integrity of judicial processes.
Personalized medicine is an exciting frontier made possible by DNA technology, employing genetic information to tailor medical treatments to individual patients. By understanding a patient’s unique genetic makeup, healthcare providers can devise more effective, targeted therapies that offer better outcomes while minimizing adverse effects. This approach not only enhances the efficacy of treatments but also paves the way for advancements in preventive medicine.
Through these applications, DNA technology continues to shape the landscape of science and healthcare, fostering innovation and yielding benefits that impact individuals and society as a whole.
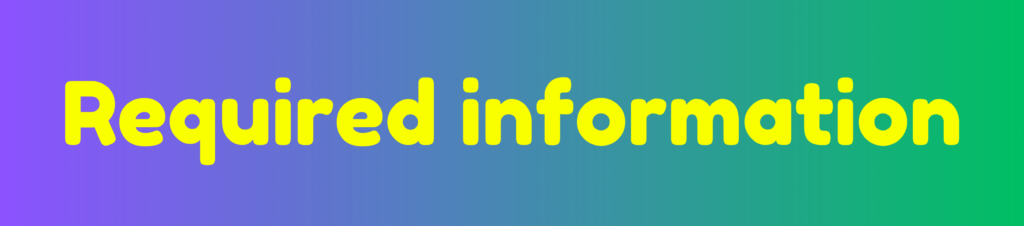
DNA in Forensic Science
The application of DNA in forensic science has revolutionized the field of criminal investigation, providing unprecedented accuracy in identifying individuals involved in criminal activities. DNA, or deoxyribonucleic acid, serves as a genetic blueprint, enabling forensic scientists to create DNA profiles that uniquely identify individuals. This process, known as DNA profiling, can be instrumental in linking a suspect to a crime scene or exonerating individuals wrongfully accused.
DNA profiling typically involves several key techniques, including polymerase chain reaction (PCR) and short tandem repeat (STR) analysis. PCR is utilized to amplify small samples of DNA, which is particularly useful in cases where only minute traces of biological material—such as hair, blood, or saliva—are available. Once the DNA is amplified, STR analysis examines specific regions where the DNA sequences vary among individuals, creating a unique pattern or “fingerprint” for each person.
Real-life case studies highlight the impact of DNA evidence on solving crimes. One notable example is the case of the Golden State Killer, a suspect linked to a string of homicides and sexual assaults in California. Investigators used DNA collected from crime scenes, comparing it to genealogical databases, which ultimately led to the identification and arrest of the suspect decades later. This case exemplifies how DNA analysis not only aids in securing convictions but also brings closure to victims’ families.
The use of DNA in forensic science underscores its importance as a powerful tool in the pursuit of justice. As technology advances, the capabilities and applications of DNA analysis will continue to evolve, further enhancing its role in crime scene investigations and the criminal justice system as a whole. The accuracy and reliability of DNA evidence remain critical in addressing the complexities of modern law enforcement.
Ethical Considerations in DNA Research
As the capabilities of DNA research and technology continue to expand, ethical implications have come to the forefront of scientific discourse. One of the most pressing concerns is genetic privacy. The ability to sequence and analyze genetic material can lead to invaluable insights into individual health and predispositions to certain conditions. However, this capability raises questions about who has access to genetic information and how it is utilized. In a world where employers, insurance companies, and even governments may seek this information, the potential for misuse looms large, threatening individual privacy and autonomy.
Alongside privacy, there is the danger of discrimination based on genetic information. Inadequate regulations may allow for individuals to face bias in hiring or receiving healthcare based on their genetic predispositions. This becomes especially problematic in contexts where individuals could be unfairly judged or treated differently due to factors beyond their control. As our understanding of genetics deepens, it is crucial to implement robust legal frameworks that protect individuals from such injustices.
Another area of ethical concern is the practice of gene editing, particularly technologies like CRISPR. While gene-editing holds great promise for curing genetic disorders, it also poses significant ethical challenges. The potential for “designer babies,” where genetic characteristics are selected based on parental preferences, opens a Pandora’s box of social and ethical dilemmas. Issues of consent, equity, and long-term effects on genetic diversity are among the primary debates within the scientific community. Researchers must navigate the fine line between innovation and ethical responsibility, ensuring that advancements do not lead to unintended consequences for individuals and society as a whole.
FAQs About DNA
DNA, or deoxyribonucleic acid, is a molecule that contains the genetic instructions for the development and function of living organisms. It serves as the blueprint of life, but many people have questions about its structure and function. Below, we address several frequently asked questions concerning DNA.
What is the structure of DNA?
DNA is structured as a double helix, which resembles a twisted ladder. This structure consists of two long strands made up of nucleotides, each containing a phosphate group, a sugar molecule, and a nitrogenous base. The four bases in DNA—adenine, thymine, cytosine, and guanine—pair specifically (adenine with thymine and cytosine with guanine), holding the strands together through hydrogen bonds.
What is the function of DNA?
DNA functions primarily to store and transmit genetic information. This information is utilized during various biological processes, including protein synthesis, which is crucial for cell function and regulation. Through the mechanisms of transcription and translation, the information encoded in DNA is converted into proteins that carry out essential tasks in the body.
How is DNA technology used today?
Advances in DNA technology, such as polymerase chain reaction (PCR) and next-generation sequencing, have revolutionized fields such as medicine, forensics, and anthropology. These techniques allow scientists to analyze genetic material with incredible precision, enabling developments in personalized medicine, genetic editing, and even ancestral DNA testing.
Are there misconceptions about DNA?
A common misconception is that DNA solely determines an individual’s traits. While DNA provides the instructions, environmental factors also play a significant role in shaping an organism’s characteristics. Additionally, there is a widespread belief that genetic alterations always result in tangible changes, which is not always the case.
By addressing these common inquiries, we hope to clarify the complexities surrounding DNA and enhance understanding of its pivotal role in biology.
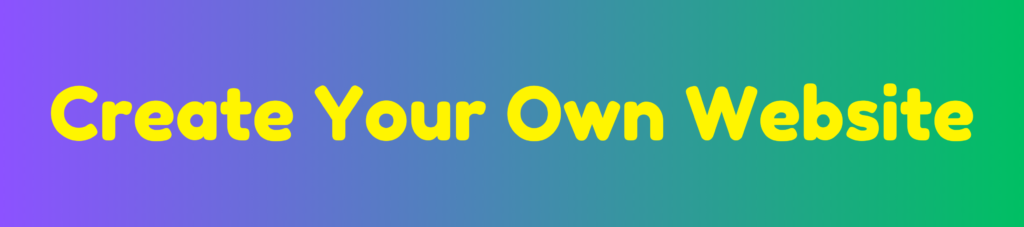
Discover more from HUMANITYUAPD
Subscribe to get the latest posts sent to your email.