Exploring DNA: The Molecule That Holds Life’s Secrets
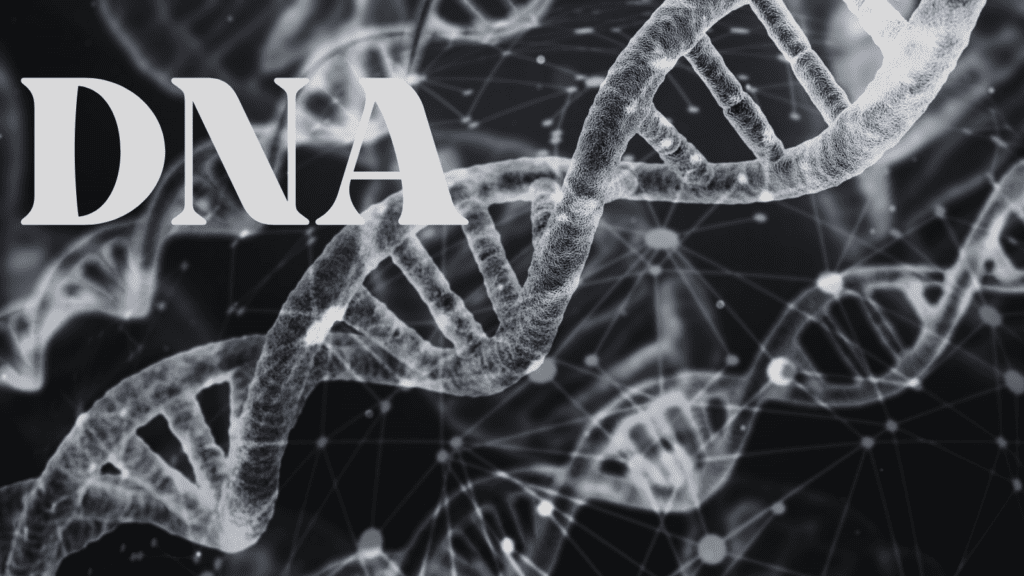
DNA, the magnificent molecule that holds the secrets of life, has fascinated scientists and researchers for centuries. Deoxyribonucleic acid, or DNA, is the fundamental building block of all living organisms, shaping our traits, behaviors, and even our susceptibility to diseases. In this blog post, we will embark on a captivating journey into the intricate world of DNA, exploring its structure, functions, and the groundbreaking discoveries that have revolutionized genetics.
The Discovery of DNA: A Historical Perspective
The discovery of DNA, a molecule that serves as the blueprint for all living organisms, is a story of scientific inquiry, collaboration, and groundbreaking revelations. The historical perspective of DNA’s discovery is a tale that spans centuries and involves the work of several brilliant scientists. Here is a brief overview:
- Prelude – The Cell Theory (17th Century): The journey to discover DNA began with the early observations of cells. In 1665, English scientist Robert Hooke used a primitive microscope to examine a thin slice of cork and coined the term “cell.” This marked the first step toward understanding the basic unit of life.
- Early Insights into Heredity (19th Century): The notion of heredity, the passing of traits from one generation to the next, was a central theme in the study of life. Gregor Mendel’s work on pea plants in the mid-19th century laid the foundation for modern genetics by establishing the principles of inheritance, although he did not know about DNA.
- Discovery of Nucleic Acids (19th Century): In the 1860s, Swiss scientist Friedrich Miescher isolated a substance from the nuclei of cells, which he called “nuclein.” He later recognized that nuclein was composed of both protein and an acidic substance he called “nucleic acid.”
- Rosalind Franklin and Maurice Wilkins (1950s): In the 1950s, British biophysicist Rosalind Franklin and her colleague Maurice Wilkins at King’s College London conducted pioneering X-ray crystallography experiments that produced high-resolution images of DNA fibers. Franklin’s famous “Photo 51” provided crucial evidence of DNA’s double-helix structure.
- Watson and Crick’s Double Helix Model (1953): James Watson, an American biologist, and Francis Crick, an English physicist, working at the University of Cambridge, used the data from Franklin’s work and combined it with their own insights. In 1953, they proposed the double-helix model of DNA, which described its structure as a twisted ladder with complementary base pairs.
- Nobel Prize and DNA’s Recognition: In 1962, James Watson, Francis Crick, and Maurice Wilkins were awarded the Nobel Prize in Physiology or Medicine for their groundbreaking discovery of the DNA structure. Unfortunately, Rosalind Franklin had passed away by then and was ineligible for the Nobel Prize.
- The DNA Revolution (20th Century and Beyond): The discovery of DNA’s structure marked the beginning of a scientific revolution. It not only led to a deeper understanding of genetics but also opened the door to the field of molecular biology, DNA sequencing, and the Human Genome Project.
The Structure of DNA: Nature’s Masterpiece
The structure of DNA, often referred to as “nature’s masterpiece,” is one of the most iconic and elegant discoveries in the history of science. Understanding the intricate structure of DNA is fundamental to comprehending the way genetic information is stored, replicated, and passed from one generation to the next. Here’s an exploration of the structure of DNA and why it is considered a masterpiece of nature:
1. The Double Helix: DNA’s most recognizable feature is its double-helix structure. Imagine a twisted ladder or spiral staircase. This double helix consists of two long chains of nucleotides that run in opposite directions. These chains are held together by hydrogen bonds between complementary base pairs.
2. Nucleotide Building Blocks: DNA is composed of building blocks known as nucleotides. Each nucleotide consists of three components: a sugar molecule (deoxyribose), a phosphate group, and one of four nitrogenous bases. The four bases are adenine (A), thymine (T), guanine (G), and cytosine (C).
3. Base Pairing: Adenine (A) always pairs with thymine (T), and guanine (G) always pairs with cytosine (C). This base pairing is a crucial feature of the DNA structure. It allows DNA to replicate accurately and forms the basis for the genetic code.
4. Antiparallel Strands: In the DNA double helix, the two chains run in opposite directions. One strand runs 5′ to 3′, while the other runs 3′ to 5′. This antiparallel arrangement is essential for DNA replication and the formation of complementary base pairs.
5. Complementary Base Pairs: The complementary base pairing ensures the specificity of the DNA structure. Adenine pairs with thymine via two hydrogen bonds, while guanine pairs with cytosine through three hydrogen bonds. This base-pairing specificity maintains the integrity of the genetic code.
6. Sugar-Phosphate Backbone: The sugar and phosphate molecules form a backbone that runs along the outside of the DNA double helix. This backbone provides structural support and protection to the delicate base pairs inside.
7. Twisting and Coiling: The DNA molecule is not simply a straight ladder; it is tightly twisted and coiled into a compact structure. This coiling allows for the efficient storage of genetic information in the cell’s nucleus.
8. Replication and Transcription: The DNA structure plays a pivotal role in DNA replication and transcription. During replication, the two DNA strands separate, and each serves as a template for the synthesis of a new complementary strand. In transcription, a single strand of DNA is used as a template to generate a complementary RNA molecule.
9. The Blueprint of Life: DNA is often referred to as the “blueprint of life” because it contains the genetic information necessary for the development, functioning, and reproduction of all living organisms. The sequence of base pairs in DNA encodes the instructions for building and maintaining an organism.
10. The Beauty of Simplicity: What makes DNA’s structure a true masterpiece is its simplicity and efficiency. The elegance of the double helix and complementary base pairing is a testament to nature’s ability to design complex systems with remarkable simplicity.
Functions of DNA: The Blueprint of Life
DNA, often referred to as the “blueprint of life,” serves as a remarkable molecule with a multitude of essential functions. Its role as the carrier of genetic information is central to understanding the development, functioning, and reproduction of all living organisms. Here, we delve into the functions of DNA and its critical role in the processes of life:
1. Genetic Information Storage: Perhaps the most fundamental function of DNA is to store genetic information. Within its sequence of base pairs, DNA encodes the instructions for building and maintaining an organism. This information includes details about an organism’s traits, characteristics, and metabolic processes.
2. DNA Replication: DNA is capable of self-replication. When a cell divides, it must create an identical copy of its DNA to pass on to the new daughter cells. DNA replication is a precise process that ensures genetic continuity. During replication, the double-stranded DNA molecule unwinds, and each strand serves as a template for the synthesis of a new complementary strand. This results in two identical DNA molecules, each with one old and one new strand.
3. Transcription: DNA serves as a template for the synthesis of another essential molecule, RNA (ribonucleic acid), through a process called transcription. During transcription, a segment of DNA is “read” by an enzyme called RNA polymerase, which synthesizes an RNA molecule complementary to the DNA template. The resulting RNA molecule, known as messenger RNA (mRNA), carries the genetic information from the DNA to the ribosome for protein synthesis.
4. Protein Synthesis: DNA indirectly controls the synthesis of proteins, which are the workhorses of the cell. In the process of protein synthesis, the information encoded in DNA is transcribed into mRNA, and then translated into a sequence of amino acids, the building blocks of proteins. This synthesis is a complex, highly regulated process that underlies every biological function in the body.
5. Gene Regulation: DNA plays a crucial role in regulating the activity of genes. Not all genes are active all the time; instead, they are switched on or off in response to the needs of the cell or organism. Various mechanisms, including the binding of proteins to specific DNA sequences, control gene expression.
6. Genetic Diversity: DNA is responsible for genetic diversity in populations. Through processes such as genetic recombination and mutations, DNA introduces variability within species, enabling adaptation to changing environments and driving evolution.
7. Inheritance: DNA is the vehicle of inheritance, passing genetic information from one generation to the next. Each offspring inherits a combination of DNA from its parents, creating unique genetic characteristics.
8. Repair and Maintenance: DNA contains a suite of repair mechanisms that help maintain its integrity. These mechanisms correct errors that may occur during replication, repair DNA damage caused by environmental factors (e.g., radiation or chemicals), and prevent mutations.
9. Telomeres and Aging: DNA contains specialized regions called telomeres at the ends of chromosomes. These structures protect genetic information during cell division. Telomere length is associated with cellular aging, and the maintenance of telomeres is a topic of research in the field of aging and longevity.
10. Epigenetic Regulation: While the DNA sequence remains relatively stable over a lifetime, epigenetic modifications, such as DNA methylation and histone modification, play a role in regulating gene expression without altering the underlying DNA sequence. These modifications can be influenced by environmental factors and have profound effects on an organism’s development and health.
The Human Genome Project: Decoding the Human Blueprint
The Human Genome Project (HGP) stands as one of the most significant scientific endeavors in history, representing a collaborative effort to decode the entire genetic blueprint of a human being. Launched in 1990 and completed in 2003, the HGP marked a monumental achievement in genomics, providing invaluable insights into human biology, medicine, and evolution. Here’s an overview of the Human Genome Project and its impact on the understanding of the human genetic code:
1. Project Initiation: The Human Genome Project was initiated by the United States National Institutes of Health (NIH) and the Department of Energy (DOE) with the goal of mapping and understanding all the genes of the human species. International collaboration, involving scientists from various countries, was a key feature of the project.
2. Mapping and Sequencing the Genome: One of the primary objectives of the HGP was to determine the sequence of nucleotide base pairs that make up human DNA and to map the genes present in the genome. Scientists used advanced technologies, including automated DNA sequencing machines, to read the sequence of DNA bases (adenine, guanine, cytosine, and thymine) in the human genome.
3. Completion of the Genome: The HGP was officially completed in 2003, two years ahead of schedule. The project’s completion marked a significant milestone, providing a high-quality, nearly complete sequence of the human genome. It was a monumental achievement in the field of genetics, representing the first draft of the human genetic code.
4. Key Discoveries and Implications:
a. Genes and Proteins: The HGP identified approximately 20,000-25,000 protein-coding genes in the human genome. These genes provide instructions for the synthesis of proteins, which are essential for the structure and function of cells.
b. Non-Coding DNA: The project revealed that only a small fraction of the genome (around 2%) encodes proteins. The rest of the genome consists of non-coding regions, some of which play regulatory roles in gene expression and other cellular processes.
c. Genetic Variation: The HGP identified millions of single nucleotide polymorphisms (SNPs) – single-letter differences in the DNA sequence – providing valuable information about genetic variation within the human population.
d. Disease Genes: Knowledge gained from the HGP has facilitated the discovery of genes associated with various diseases, enabling advancements in diagnostics, treatment, and drug development. It laid the foundation for the field of personalized medicine.
5. Ethical, Legal, and Social Implications (ELSI): The HGP also recognized the importance of addressing the ethical, legal, and social implications of genomic research. A portion of the project’s funding was allocated to studying these issues, leading to the development of guidelines and policies for genetic research and data privacy.
6. Post-Genomic Era: Following the completion of the HGP, the field of genomics entered the post-genomic era. Continued research focused on understanding the functions of genes, unraveling complex gene interactions, studying epigenetic modifications, and exploring the roles of non-coding RNAs, among other areas of genomic investigation.
DNA Technology: Transforming Science and Society
DNA technology, also known as genetic engineering or biotechnology, has transformed science and society in profound ways. This field of study and practice revolves around the manipulation, analysis, and utilization of DNA molecules. It has a far-reaching impact on various sectors, including medicine, agriculture, forensics, and environmental conservation. Here, we explore the role of DNA technology in transforming both science and society:
1. Advancements in Medicine:
a. Genomic Medicine: DNA technology has paved the way for personalized medicine, where treatments and medications can be tailored to an individual’s genetic makeup. This approach is especially promising in cancer treatment, rare diseases, and pharmacogenomics.
b. Gene Therapy: Gene therapy uses DNA technology to correct or replace faulty genes, offering potential cures for genetic diseases. It is a burgeoning field with applications in inherited disorders, immune system disorders, and certain types of cancer.
c. DNA Sequencing: The development of high-throughput DNA sequencing techniques has revolutionized healthcare. It allows for the rapid and cost-effective analysis of an individual’s entire genome, leading to better diagnoses and treatment strategies.
2. Agriculture and Food Production:
a. Genetically Modified Organisms (GMOs): DNA technology has enabled the creation of GMOs, which possess specific genetic traits, such as resistance to pests, improved nutritional content, or tolerance to environmental stress. These modified crops have the potential to increase food production and reduce the need for chemical pesticides.
b. Crop Improvement: Genetic engineering has the potential to enhance crop yields and develop plants with desirable traits, such as drought resistance, which is crucial for global food security.
3. Forensics and Criminal Justice:
a. DNA Profiling: DNA technology has revolutionized forensics by enabling DNA profiling, which is used in criminal investigations and identifying individuals through DNA fingerprinting. It has greatly improved accuracy in criminal justice and legal proceedings.
b. Cold Cases and Exonerations: DNA analysis has played a crucial role in solving cold cases and exonerating individuals wrongfully convicted of crimes. It has shed light on the reliability of eyewitness accounts and other forms of evidence.
4. Environmental Conservation:
a. Environmental DNA (eDNA): Scientists use eDNA to detect and monitor species in their natural habitats. This technology aids in biodiversity conservation and ecosystem management.
b. De-extinction: DNA technology is explored in “de-extinction” efforts, where scientists aim to revive extinct species by using preserved DNA samples.
5. Gene Editing and Synthetic Biology:
a. CRISPR-Cas9: The development of the CRISPR-Cas9 gene-editing technology has made precise modifications to DNA sequences easier and more accessible. It has applications in basic research, agriculture, and human health.
b. Synthetic Biology: DNA technology is at the heart of synthetic biology, where researchers engineer biological systems and design new organisms for various purposes, such as bioremediation, biofuel production, and drug synthesis.
6. Ethical and Societal Implications:
a. Ethical Dilemmas: DNA technology has raised numerous ethical questions, including concerns about genetic privacy, designer babies, and the unintended consequences of genetic modifications.
b. Legal and Regulatory Frameworks: The development of DNA technology has necessitated the establishment of legal and regulatory frameworks to ensure responsible and ethical use in various applications.
Conclusion
The story of DNA is a remarkable saga of scientific curiosity, collaboration, and innovation. From the early observations of cells to the groundbreaking discovery of the DNA double helix and the completion of the Human Genome Project, humanity’s understanding of DNA has paved the way for transformative advancements in genetics, medicine, and technology.
As we continue to unravel the mysteries of DNA and explore its myriad applications in fields such as medicine, agriculture, forensics, and environmental conservation, it is crucial to navigate the ethical and societal implications associated with these advancements. The power of DNA technology, while awe-inspiring, comes with great responsibility. Striking a balance between scientific progress and ethical considerations is essential to harness the full potential of DNA technology for the betterment of society.
In this ever-evolving landscape of genetic discovery, one thing remains certain: DNA, the magnificent molecule that holds the secrets of life, will continue to inspire scientists, researchers, and society as a whole, driving us toward a future where the potential of this extraordinary molecule is harnessed for the greater good of humanity.
Disclaimer
This blog post is intended for informational purposes only. It provides an overview of DNA, its historical discovery, structure, functions, the Human Genome Project, and DNA technology. It does not constitute medical, legal, or ethical advice. For specific concerns or questions related to DNA and its applications, readers are encouraged to consult with qualified professionals or experts in the respective fields.
Stay updated—subscribe now for informed empowerment!