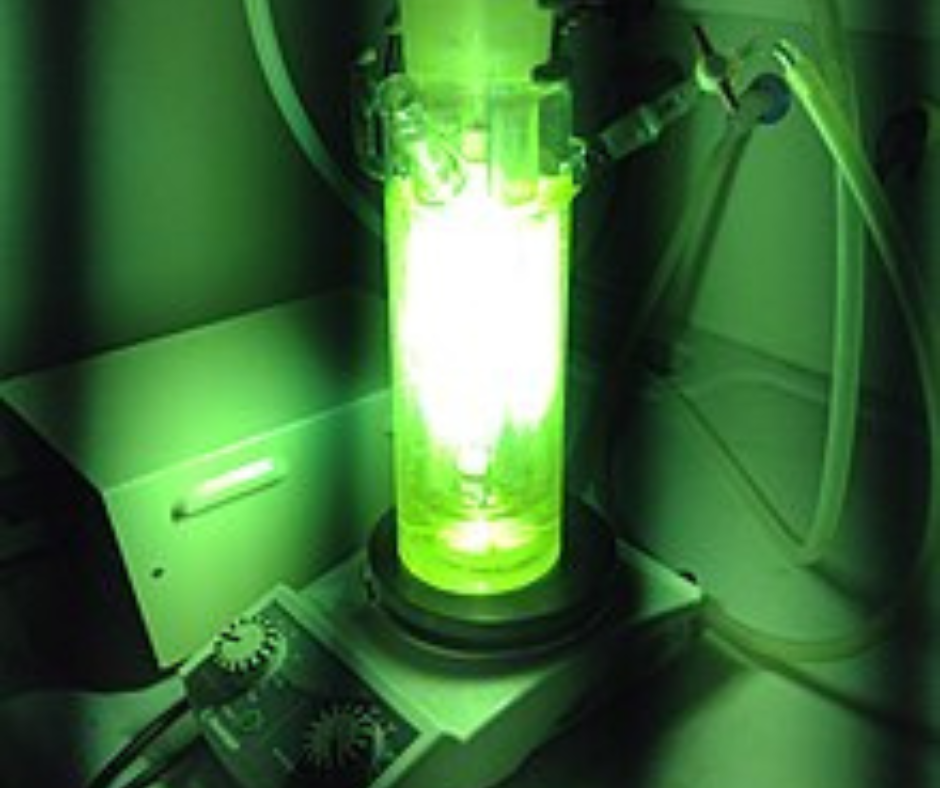
Introduction to Photochemistry
Photochemistry is a branch of chemistry that studies the chemical reactions and processes that are initiated or driven by light. The interaction of light with matter gives rise to a myriad of chemical phenomena, where photons excite electrons, initiating various reactions. This field is significant as it encompasses a wide range of applications, from understanding the mechanisms of photosynthesis in plants to developing innovative technologies such as solar energy conversion and phototherapeutic techniques in medicine.
The basic principles of photochemical processes revolve around the absorption of light and the energy transfer that occurs subsequently. When a molecule absorbs a photon, its electrons become energized, moving from a ground state to an excited state. This transition can result in chemical changes, such as breaking bonds or forming new ones. Unlike traditional chemistry, where reactions are predominantly driven by thermal energy, photochemical reactions rely on light energy, introducing additional complexities to reaction mechanisms and pathways.
Historically, photochemistry has undergone substantial development. The groundwork was laid in the early 19th century with the discovery of the photoelectric effect by Heinrich Hertz, which was further advanced by Albert Einstein, establishing a deeper understanding of light’s properties. Subsequent advancements in the understanding of ultraviolet (UV) light and its interaction with chemical species, such as in the work of R. G. Kauffman and A. A. C. S. de Lima, have fortified the foundations of this discipline. Moreover, the discovery of photosensitive materials and phenomena, such as fluorescence and phosphorescence, have influenced various fields including biochemistry and material science. As a result, photochemistry not only enriches our comprehension of chemical processes but also inspires technological advancements essential for future innovations.
Key Principles of Photochemistry
Photochemistry is a branch of chemistry focused on the interaction between light and matter, particularly how photons influence chemical reactions. One essential principle involves the absorption of light, where molecules capture energy from photons, promoting electrons to higher energy levels. This process is foundational as it initiates subsequent chemical reactions. The absorbed light must match the specific excitation wavelengths of the atoms or molecules; otherwise, the interaction will not occur effectively. Each molecule has unique energy levels characteristic of its structure, which determines which wavelengths it can absorb.
Once a molecule absorbs a photon, it transitions to an excited state. This state is categorized into different types—most notably, singlet and triplet states. A singlet state occurs when the spins of the excited electron and unimpaired electron pair are opposite, resulting in a net zero spin. Conversely, in a triplet state, the spins are parallel, leading to a net spin state of one. This distinction is vital as it affects the molecule’s reactivity and the pathways available for subsequent reactions. The lifetime of the excited state also varies, influencing whether the molecule will return to its ground state through non-radiative processes or emit light through fluorescence.
Another fundamental aspect of photochemistry is the quantum yield, which quantifies the efficiency of a photochemical reaction. It is defined as the ratio of the number of photochemical events to the number of photons absorbed. A high quantum yield indicates a highly efficient process, whereas a low yield suggests that many absorbed photons do not lead to a chemical change. Understanding these principles enables researchers to design and optimize photochemical processes, influencing applications ranging from solar energy conversion to medicinal chemistry.
Common Photochemical Reactions
Photochemical reactions encompass a diverse range of processes that are driven by the absorption of light. Among the most well-known of these reactions is photosynthesis, a fundamental biochemical process utilized by green plants, algae, and some bacteria. In photosynthesis, chlorophyll captures sunlight, facilitating the conversion of carbon dioxide and water into glucose and oxygen. This process not only provides energy for the organisms involved but also plays a crucial role in supporting life on Earth by producing oxygen and serving as the foundation of the food web.
Another significant type of photochemical reaction is photooxidation. This process involves the reaction of a substance with oxygen in the presence of light, resulting in the formation of reactive oxygen species. A practical example of photooxidation is the degradation of pollutants in environmental remediation. Ultraviolet (UV) light can be employed to activate certain catalysts, enhancing the breakdown of harmful substances in water bodies, thus promoting detoxification and improving water quality.
Polymerization is also a noteworthy photochemical reaction, particularly in the field of industrial applications. In photopolymerization, light is utilized to initiate chemical reactions that form polymers from monomers. This mechanism is extensively employed in making coatings, adhesives, and 3D printing materials. The process is favored for its efficiency and precision, allowing manufacturers to control the properties of the final product by fine-tuning light exposure. The development of photoinitiators, which absorb light and generate reactive species, has further enhanced this technique, offering a wide range of applications across various industries.
Understanding these common photochemical reactions is essential for harnessing their potential in both natural ecosystems and industrial processes. Each reaction exemplifies the intricate interplay between light and matter, highlighting the importance of photochemistry in our daily lives.
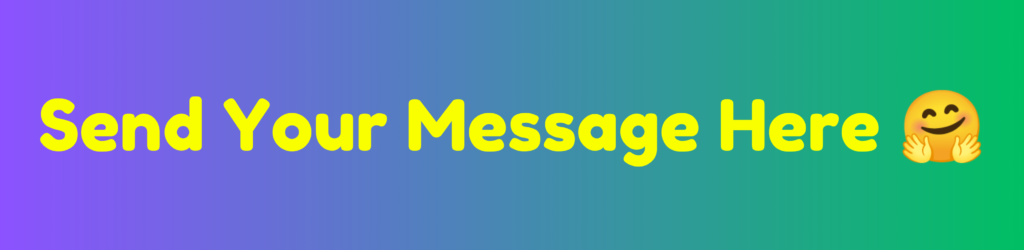
Applications of Photochemistry
Photochemistry encompasses a wide array of applications across various fields, demonstrating its significant role in driving innovation and addressing contemporary challenges. In environmental science, photochemical processes are leveraged for solar energy conversion, whereby sunlight is harnessed to generate power through photovoltaic cells and chemical reactions. This method is fundamental in the development of renewable energy solutions aimed at reducing reliance on fossil fuels and combating climate change. Solar energy systems not only enhance energy efficiency but also offer a sustainable alternative to traditional energy sources.
In the medical field, phototherapy has emerged as a valuable treatment option for a variety of conditions. This technique utilizes light to trigger photochemical reactions within the body, aiding in the healing process. For example, blue light therapy is commonly employed in the treatment of acne, while photodynamic therapy is used to target cancer cells. These methods effectively demonstrate how photochemistry plays a crucial role in enhancing treatment efficacy and patient outcomes, thus emphasizing its relevance in advancing healthcare technologies.
Moreover, the development of innovative materials through photochemical processes has opened new avenues in materials science. Photoinitiators are substances that absorb light to initiate polymerization, leading to the formation of new materials with unique properties. This approach is applied in the creation of coatings, adhesives, and 3D printing materials, illustrating the versatility of photochemistry in engineering applications. The ongoing research in this field aims to further enhance the performance and sustainability of materials, aligning with environmental stewardship goals.
Case studies highlight the importance of photochemistry in tackling real-world challenges. For instance, the application of photocatalysts in water purification systems is a promising method for removing contaminants, showcasing the intersection of photochemistry and environmental sustainability. Such examples underscore the transformative potential of photochemical processes across various sectors, reinforcing its vital role in the modern world.
Photochemistry in Environmental Science
Photochemistry plays a critical role in various environmental processes, significantly impacting air quality, water purification, and climate dynamics. The natural phenomenon of photochemical reactions involves the absorption of light, leading to chemical transformations that are essential for the breakdown of pollutants in the environment. These reactions are particularly important in understanding how pollutants interact with sunlight, resulting in the formation of secondary pollutants that can adversely affect air quality.
One of the most notable instances of photochemistry in atmospheric science is the formation of ozone (O3) in the troposphere. This process begins when sunlight triggers photochemical reactions involving volatile organic compounds (VOCs) and nitrogen oxides (NOx). During these reactions, ozone is formed as a secondary pollutant, contributing to the greenhouse effect and posing risks to human health and the environment. Understanding the photochemical pathways that lead to ozone formation is vital for developing effective air quality management strategies.
Beyond atmospheric chemistry, photochemistry also plays an essential role in water treatment processes. Photochemical oxidation methods, such as advanced oxidation processes (AOPs), utilize light to generate reactive species capable of decomposing organic pollutants in wastewater. These techniques have proven effective in degrading persistent contaminants, thus enhancing water quality and ensuring safer drinking water supplies. Moreover, photochemical processes are involved in the natural purification of water bodies, where sunlight initiates the breakdown of harmful substances by facilitating molecular transformations.
Additionally, photochemical reactions contribute to the cycling of vital elements such as carbon and nitrogen within ecosystems. For instance, photosynthesis, a crucial photochemical process, enables plants to convert solar energy into chemical energy, supporting the food chain. Moreover, nitrogen fixation processes involve light-induced reactions that convert atmospheric nitrogen into forms usable by living organisms, demonstrating the interconnections between photochemistry and ecosystem health.
Challenges in Photochemistry Research
Photochemistry, the study of chemical reactions initiated by light, presents an array of challenges that researchers are continually striving to overcome. One of the foremost complexities arises from the intricate nature of photochemical processes themselves. The interactions between light and matter are often non-linear and can lead to the formation of multiple products, complicating both theoretical understanding and practical applications. As a result, predicting reaction outcomes accurately becomes a formidable task, necessitating sophisticated models that account for various factors such as energy transfer, molecular dynamics, and environmental influences.
Another significant hurdle is the difficulty in modeling these complex photochemical reactions. Traditional computational chemistry methods can struggle to provide satisfactory accuracy for systems where light-induced reactions lead to rapid changes in molecular structures. This challenge highlights the urgent need for novel computational strategies that can incorporate real-time kinetic and thermodynamic data. Furthermore, the transition states in photochemical reactions are often short-lived, which adds another layer of complexity to modeling efforts. Researchers are thus focusing on developing enhanced modeling techniques that may include machine learning algorithms to predict reaction pathways more reliably.
Additionally, the advancement of experimental techniques is pivotal for overcoming these challenges. For instance, the development of ultrafast spectroscopy has opened new avenues for probing transient species formed during photochemical reactions. Innovative methods, such as time-resolved imaging, allow researchers to capture and analyze reaction intermediates on an unprecedented timescale. Despite these developments, the realization of capturing every nuance in photochemical systems remains elusive. The implications of these challenges extend beyond mere scientific inquiry; they impact the advancement of technologies reliant on photochemistry, such as solar energy conversion and photodynamic therapy, making it crucial to address these issues through interdisciplinary approaches and collaborative research efforts.
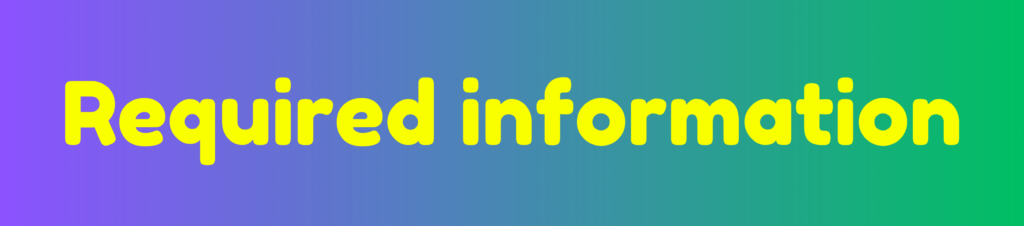
Future Trends in Photochemistry
The field of photochemistry is poised for remarkable advancements that will reshape our understanding and application of light-driven chemical processes. Among the emerging trends, the development of photonic materials stands out as a significant area of focus. These materials, which manipulate and interact with light in novel ways, are expected to enhance the efficiency and versatility of photochemical reactions. Innovations such as metamaterials and nanostructured surfaces are paving the way for improved light absorption and better energy transfer mechanisms, potentially leading to breakthroughs in photochemical efficiency.
Another promising avenue presents itself through the integration of photochemistry with artificial intelligence (AI). The utilization of AI technologies facilitates the optimization of photochemical processes by analyzing vast datasets, predicting reaction outcomes, and fine-tuning experimental conditions. Machine learning algorithms can help identify new photochemical pathways, allowing researchers to explore previously uncharted territories in molecular interactions and reaction kinetics. This symbiotic relationship between photochemistry and AI is likely to accelerate the pace of discovery and innovation in the field.
Additionally, sustainable energy solutions are becoming increasingly intertwined with photochemical principles. The ongoing quest for renewable energy sources has led to the development of photochemical systems that can efficiently convert solar energy into chemical fuels. This includes processes like artificial photosynthesis, which aims to mimic the natural conversion of sunlight into energy by plants. With growing environmental concerns and the imperative to transition to sustainable resources, advancements in photochemical technology will play a crucial role in addressing global energy challenges.
Experts predict that the coming years will see an explosion of new discovery and application opportunities in photochemistry. As research continues to evolve and interdisciplinary collaborations flourish, the field will undoubtedly witness exciting innovations that will enhance our capacity to harness light for various practical applications.
Frequently Asked Questions about Photochemistry
Photochemistry is a fascinating field that often raises numerous questions among students, researchers, and industry professionals alike. This section aims to provide clear and concise answers to some of the most frequently asked questions related to this science.
What is photochemistry?
Photochemistry is the study of chemical reactions, processes, and changes that result from the interaction of light with matter. It encompasses the understanding of how light energy influences chemical transformations, such as the formation and breaking of chemical bonds, which can lead to new substances. This field is essential for understanding various natural and artificial processes, from photosynthesis to solar energy conversion.
How does light affect chemical reactions?
Light, being a form of electromagnetic radiation, can excite electrons in atoms and molecules to higher energy states. This energy input can then provoke specific chemical reactions. In photochemical reactions, the absorbed light energy can lead to the formation of reactive intermediates, which can subsequently produce a range of products through further reactions. This mechanism is utilized in various applications, including photovoltaic cells and phototherapy in medicine.
What are some practical applications of photochemistry?
Photochemistry finds applications in numerous fields, including environmental science, energy production, and the pharmaceutical industry. For example, it plays a crucial role in the development of solar energy technologies, where it assists in converting sunlight into usable energy through photovoltaic systems. Furthermore, photochemistry is integral to processes such as photopolymerization in the production of plastics and UV curing in coatings.
Are there any environmental implications related to photochemistry?
Yes, photochemical processes can have both beneficial and detrimental effects on the environment. On one hand, photochemistry contributes to the breakdown of pollutants in the atmosphere through photolysis, aiding in air purification. Conversely, certain photochemical reactions can lead to the formation of harmful by-products, such as ground-level ozone, which poses health risks and contributes to climate change.
What is the role of photons in photochemistry?
Photons are packets of light energy that initiate photochemical reactions. When a molecule absorbs a photon, it transitions to an excited state, increasing its energy. This excitation often makes the molecule more reactive, enabling it to undergo chemical transformations that would not occur under normal conditions.
What is the difference between photochemistry and thermochemistry?
Photochemistry involves chemical reactions driven by light energy, while thermochemistry focuses on reactions influenced by heat. The primary distinction lies in the energy source: photochemistry uses photons, whereas thermochemistry relies on thermal energy to overcome activation barriers.
What are some common photochemical reactions?
Some common photochemical reactions include:
- Photosynthesis: Plants convert carbon dioxide and water into glucose and oxygen using sunlight.
- Photodegradation: Breakdown of materials like plastics or pollutants under UV light.
- Photoisomerization: Light-induced structural changes in molecules, as seen in vision (e.g., rhodopsin isomerization in the eye).
- Photopolymerization: Formation of polymers from monomers using light, used in dental fillings and 3D printing.
How is photochemistry used in medicine?
Photochemistry has various medical applications, including:
- Photodynamic therapy (PDT): Treating cancer and skin disorders by activating photosensitizers with light.
- UV sterilization: Using ultraviolet light to kill bacteria and viruses.
- Drug development: Designing light-sensitive drugs for controlled release and activation in specific areas of the body.
What safety precautions are necessary for photochemical experiments?
Conducting photochemical experiments requires careful safety measures to prevent accidents and exposure to harmful light sources:
- Wear UV-blocking goggles and protective clothing.
- Use proper shielding to contain light radiation.
- Work in well-ventilated areas to avoid accumulation of photochemically reactive by-products.
- Handle chemicals with appropriate gloves and follow safety data sheet (SDS) guidelines.
What instruments are commonly used in photochemistry?
Key instruments include:
- Spectrophotometers: Measure light absorption by molecules.
- Laser systems: Provide precise light sources for experiments.
- Photoreactors: Facilitate controlled photochemical reactions.
- Fluorimeters: Detect fluorescence emitted by excited molecules, aiding in studying reaction mechanisms.
What are photochemical intermediates, and why are they important?
Photochemical intermediates are transient species formed during photochemical reactions, such as radicals, excited states, or ions. These intermediates often determine the reaction pathway and final products. Studying them helps scientists understand reaction mechanisms and optimize processes like solar energy conversion and synthetic photochemistry.
What challenges exist in photochemistry research?
Challenges include:
- Efficiently harvesting and utilizing light energy in artificial systems.
- Minimizing undesired side reactions and by-products.
- Understanding and controlling complex photochemical reaction mechanisms.
- Scaling laboratory photochemical processes for industrial applications.
How does photochemistry play a role in solar energy conversion?
Photochemistry is central to solar energy conversion technologies like solar cells. In photovoltaic cells, light energy is absorbed by semiconductor materials, exciting electrons to generate electricity. This process is essentially a photochemical reaction, where the energy from photons is converted into usable electrical energy. Ongoing research is focused on improving the efficiency of these processes to make solar power a more sustainable energy source.
What is the impact of photochemistry on climate change?
Photochemical reactions can both mitigate and exacerbate climate change. On the positive side, photochemistry contributes to the removal of pollutants, such as carbon monoxide and volatile organic compounds, from the atmosphere through photolysis. However, certain photochemical processes, such as the formation of ground-level ozone, can contribute to air pollution and global warming. Understanding these processes is essential to finding solutions to climate-related challenges.
How are photochemical processes used in the production of materials?
Photochemistry is widely used in manufacturing processes such as photopolymerization, which helps produce plastics, coatings, and adhesives. UV light is used to initiate the polymerization of monomers, leading to solid polymers. This is a key technology in industries like printing, electronics, and medical device manufacturing. Similarly, photochemistry plays a role in creating high-quality materials like optical fibers and semiconductors.
What are the main types of photochemical reactions?
The main types of photochemical reactions include:
- Photodissociation: Breaking of bonds in molecules by the absorption of light, often seen in atmospheric chemistry.
- Photoaddition: Addition of atoms or molecules to form new compounds under light exposure.
- Photooxidation: Oxidation of substances in the presence of light, which is crucial in processes like photosynthesis and the degradation of pollutants.
What is the role of photochemistry in environmental pollution control?
Photochemistry is vital in controlling environmental pollution. Photochemical processes help break down harmful substances in the atmosphere, such as greenhouse gases and pollutants, through reactions like photolysis. Additionally, photochemistry is used in developing advanced water and air purification technologies, helping to reduce harmful chemicals in the environment.
What is the relationship between photochemistry and atmospheric chemistry?
Photochemistry and atmospheric chemistry are closely linked. Many reactions in the atmosphere, such as the formation of ozone or the degradation of pollutants, are driven by light. Understanding photochemical processes in the atmosphere is essential for predicting air quality, climate change, and the behavior of pollutants over time. Atmospheric photochemistry also plays a key role in the Earth’s natural cycles, including the water and carbon cycles.
What are the potential future developments in photochemistry?
Future developments in photochemistry may focus on improving solar energy efficiency, developing light-driven chemical processes for sustainable production, and advancing medical treatments like photodynamic therapy. Researchers are also exploring ways to harness light for more efficient industrial applications, such as green chemistry and waste recycling, using light to power chemical reactions that would otherwise require high heat or toxic chemicals.
Conclusion
Photochemistry is a rapidly advancing field with wide-reaching applications across various industries, from energy production to environmental protection and medicine. Its role in understanding and manipulating light-driven chemical reactions is indispensable for technological advancements and sustainable practices. As research continues, the applications of photochemistry will likely expand, offering solutions to some of the most pressing global challenges.
We hope this FAQ has provided you with a clearer understanding of photochemistry and its significance. If you have further questions or want to dive deeper into a particular aspect of photochemistry, feel free to explore more resources or consult experts in the field. The possibilities in photochemistry are vast, and its impact on our world will continue to grow in the coming years.
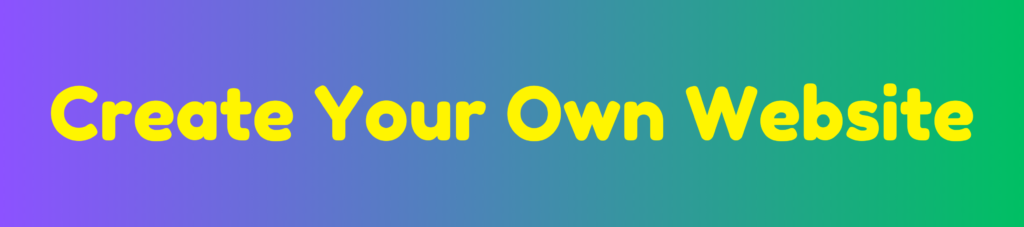
Discover more from HUMANITYUAPD
Subscribe to get the latest posts sent to your email.