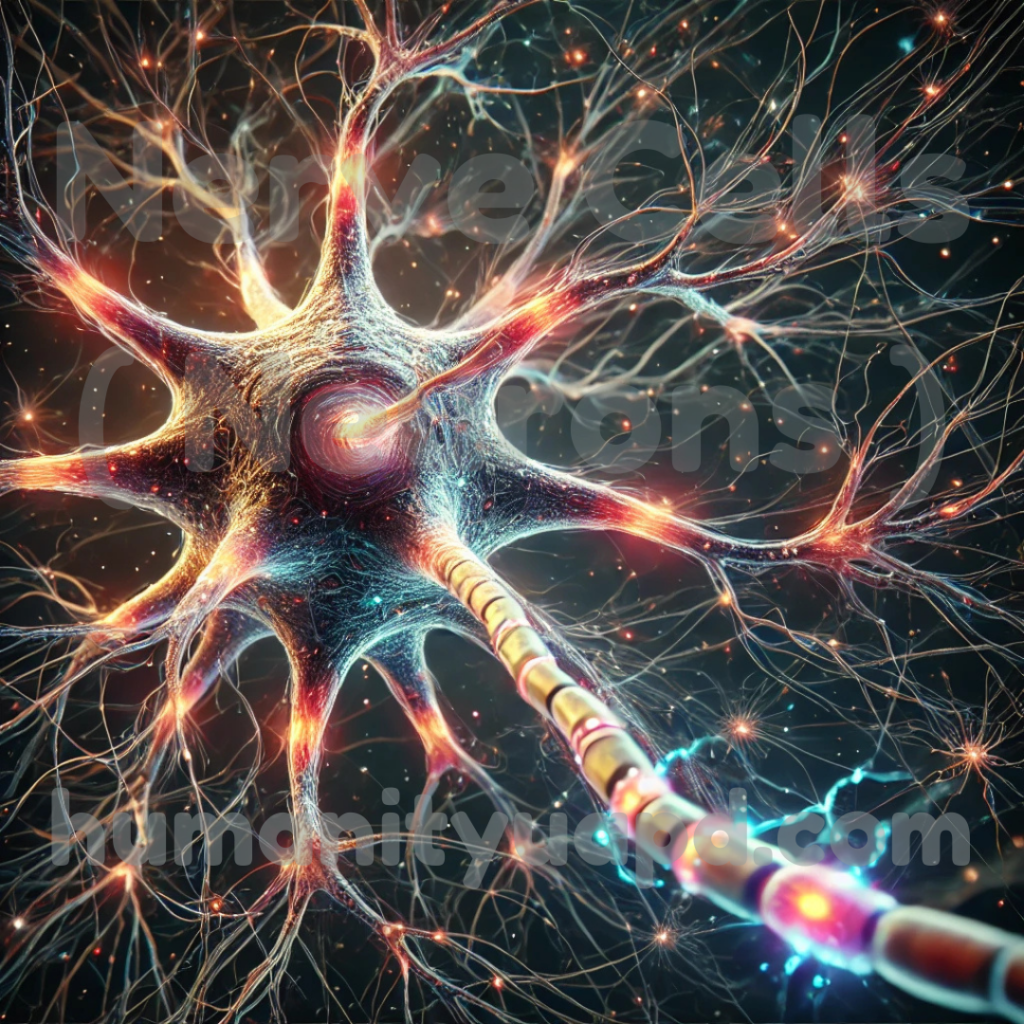
Introduction to Nerve Cells
Nerve cells, commonly known as neurons, are specialized cells that serve as the fundamental building blocks of the nervous system. They play a crucial role in transmitting information throughout the body by conducting electrical impulses and exchanging signals with other neurons, muscle cells, and gland cells. Neurons are distinct from other cell types due to their unique structure and function, which enable them to communicate rapidly and efficiently. Each neuron comprises three main parts: the cell body, dendrites, and the axon. The cell body contains the nucleus and cellular machinery, while dendrites receive incoming signals from other cells, and the axon transmits impulses away from the cell body.
The importance of nerve cells in the nervous system cannot be overstated. Neurons are essential for processing sensory information, regulating motor functions, and facilitating cognitive processes such as memory and learning. This ability to adapt and reorganize is referred to as neuroplasticity, signifying that nerve cells can modify their connections and behavior in response to experiences and environmental changes. This feature is particularly significant in rehabilitation following injuries, where neuroplasticity allows for the potential recovery of lost functions.
Unlike other cells in the body, neurons have a limited ability to regenerate after damage. This limitation highlights the crucial need to maintain neuronal health and well-being through lifestyle factors such as nutrition, physical activity, and mental stimulation. Furthermore, the study of nerve cells is essential for understanding various neurological disorders, which can arise from dysfunctions in neuronal communication, such as Alzheimer’s disease, Parkinson’s disease, and multiple sclerosis. As we delve deeper into the intricate world of nerve cells, we uncover their fundamental role in shaping our thoughts, actions, and overall quality of life.
Anatomy of Nerve Cells
Nerve cells, or neurons, are specialized cells responsible for transmitting nerve impulses throughout the body. Their intricate structure is crucial for their primary function in the nervous system, which is to facilitate communication between different body parts. The basic components of a nerve cell include the cell body, dendrites, axon, and synapses, each playing a vital role in nerve signal transmission.
The cell body, or soma, serves as the central hub of the neuron. It contains the nucleus and organelles necessary for the cell’s metabolic functions. This structure is responsible for maintaining the health of the neuron and integrating incoming signals from other neurons. Surrounding the cell body is the cytoplasm, which provides the necessary environment for cellular activities.
Dendrites are tree-like extensions that emanate from the cell body. Their primary function is to receive signals from neighboring neurons, converting these signals into electrical impulses. Dendrites increase the surface area of the neuron, allowing it to establish numerous connections with other neurons, thus amplifying its capacity to receive information.
The axon, typically a long and slender projection, extends from the neuron and serves to transmit electrical impulses away from the cell body. The myelin sheath, a fatty layer that envelops the axon, plays a critical role in accelerating the transmission of these impulses. This insulation allows for faster communication between nerve cells and enhances the efficiency of the nervous system.
At the end of the axon are synapses, the junctions through which neurons communicate with one another. When an impulse reaches the synapse, it triggers the release of neurotransmitters—chemical messengers that carry signals across the synaptic gap to the next neuron. This intricate mechanism showcases how nerve cells work together to transmit information effectively, highlighting the complexity of the nervous system’s architecture.
Types of Nerve Cells
Nerve cells, or neurons, represent essential components of the nervous system, functioning as the primary units for signal transmission. There are three main types of nerve cells: sensory neurons, motor neurons, and interneurons. Each type has specialized functions that contribute to the overall operation of the nervous system, facilitating communication and coordination throughout the body.
Sensory neurons are responsible for transmitting sensory information from various sensory organs to the central nervous system. They detect stimuli such as light, sound, touch, and chemical changes, converting these external signals into electrical impulses. For instance, when you touch a hot surface, sensory neurons convey the painful stimulus to the brain, prompting an immediate reaction to withdraw your hand, thus preventing injury.
Motor neurons, on the other hand, take the signals generated within the central nervous system and relay them to muscles, directing movements and actions. They play a pivotal role in translating cognitive decisions into physical responses. For example, when you decide to lift an object, motor neurons activate the relevant muscles to execute this action, ensuring a seamless interaction between the nervous system and the muscular system.
Interneurons serve as the connectors between sensory and motor neurons, facilitating communication within the central nervous system. They process information and relay signals between different nerve cells, allowing complex reflexes and cognitive functions to occur. Interneurons can be found within the spinal cord as well as other regions of the brain, integrating sensory input with appropriate motor output. An example of this is the reflex arc, where interneurons connect sensory inputs directly to motor outputs, enabling rapid responses without necessitating input from higher brain centers.
Each type of nerve cell plays a crucial role in ensuring the efficient functioning of the nervous system, enabling the body to respond to internal and external stimuli effectively.
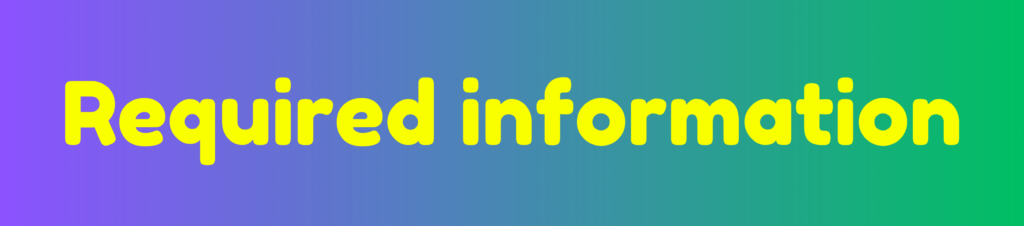
How Nerve Cells Communicate
Nerve cells, also known as neurons, are specialized cells that play a critical role in the transmission of signals throughout the nervous system. The communication between nerve cells occurs primarily through a process known as synaptic transmission. This complex interaction involves both electrical and chemical signals, enabling neurons to relay information effectively and efficiently.
At the core of synaptic transmission is the synapse, which is the junction between two neurons. When an electrical signal, known as an action potential, reaches the end of a neuron (axon terminal), it triggers the release of neurotransmitters. These chemical messengers are stored in vesicles and are crucial for conveying information. Once released into the synaptic cleft—the small gap between neurons—neurotransmitters bind to receptors on the surface of the receiving neuron, leading to various responses, such as the generation of a new action potential.
Electrical signals also play a vital role in this communication pathway. The action potential is an all-or-nothing response that involves the rapid depolarization and repolarization of the neuron’s membrane. This electrical signal travels along the axon to the axon terminal, where it ultimately initiates the release of neurotransmitters. Additionally, the communication process can be modulated by various factors, including the type of neurotransmitter released, the density of receptors on the receiving neuron, and the presence of other molecules that can enhance or inhibit signaling.
Different neurotransmitters serve distinct functions, influencing numerous physiological processes such as mood, perception, and motor control. For instance, dopamine is often associated with pleasure and reward, while serotonin plays a key role in regulating mood and social behavior. Thus, the intricate mechanisms involved in how nerve cells communicate highlight the importance of synaptic transmission in maintaining proper function within the nervous system.
The Role of Nerve Cells in the Nervous System
Nerve cells, or neurons, are fundamental components of both the central nervous system (CNS) and the peripheral nervous system (PNS), playing a critical role in information processing and communication throughout the body. In the CNS, which comprises the brain and spinal cord, neurons are integral to the processing and integration of sensory information, motor commands, and higher cognitive functions. They communicate with each other through synapses, forming complex networks that enable the execution of intricate tasks, such as movement, perception, and decision-making.
The CNS relies heavily on two primary types of nerve cells: afferent (sensory) neurons, which transmit sensory information to the brain, and efferent (motor) neurons, which convey commands from the brain to muscles and glands. Interneurons, another type found predominantly in the CNS, facilitate communication between sensory and motor neurons, allowing for reflex actions and complex behaviors. This intricate web of connections allows the nervous system to function as a cohesive unit.
In contrast, the peripheral nervous system extends beyond the CNS and includes all nerve pathways that connect the CNS to limbs and organs. Nerve cells in the PNS are crucial for transmitting signals to and from the CNS, enabling the body to react to environmental stimuli. The PNS consists of three branches: the somatic nervous system, which governs voluntary movements; the autonomic nervous system, responsible for automatic processes such as heartbeat and digestion; and the enteric nervous system, which regulates gut function.
Through their coordinated activity, nerve cells facilitate the rapid transmission of information, ensuring that the body can respond swiftly and appropriately to changes in the environment. The role of nerve cells is therefore essential, as they form the backbone of the nervous system, allowing for seamless communication and coordination across all bodily functions.
Neurodegenerative Diseases and Nerve Cells
Neurodegenerative diseases are a group of disorders characterized by the progressive degeneration of the structure and function of the nervous system, particularly affecting nerve cells or neurons. These conditions, including Alzheimer’s disease, Parkinson’s disease, and multiple sclerosis, pose significant challenges not only for the individuals diagnosed but also for their families and the healthcare system at large.
Alzheimer’s disease is notably one of the most common neurodegenerative conditions, leading to memory loss, cognitive decline, and ultimately, loss of ability to perform everyday tasks. In Alzheimer’s, the nerve cells experience the formation of amyloid plaques and tau tangles, resulting in the disruption of communication between neurons and their eventual death. This neuronal loss leads to the hallmark symptoms associated with the disease, profoundly impacting the patient’s cognitive functions.
Similarly, Parkinson’s disease primarily affects motor function and is distinguished by the gradual loss of dopamine-producing neurons in the substantia nigra, a critical area of the brain. The depletion of dopamine leads to the classic symptoms of tremors, rigidity, and bradykinesia. This progressive nature of Parkinson’s not only affects movement but also has implications for cognitive functions, mood, and overall quality of life.
Multiple sclerosis (MS), on the other hand, is an autoimmune disease where the immune system erroneously attacks the protective sheath (myelin) surrounding nerve cells. The damage to myelin results in disrupted communication between the brain and the body, leading to symptoms that can vary widely, from fatigue and weakness to impaired vision and coordination. Research is ongoing to better understand the mechanisms behind these diseases, their impact on nerve cells, and potential therapeutic interventions.
Current studies emphasize the importance of early diagnosis and treatment in managing these debilitating diseases, as well as the potential for novel therapies aimed at neuroprotection and regeneration of nerve cells. The integration of multidisciplinary approaches, including pharmacological treatments and lifestyle interventions, may enhance the quality of life for affected individuals and provide hope for future advancements in the field.
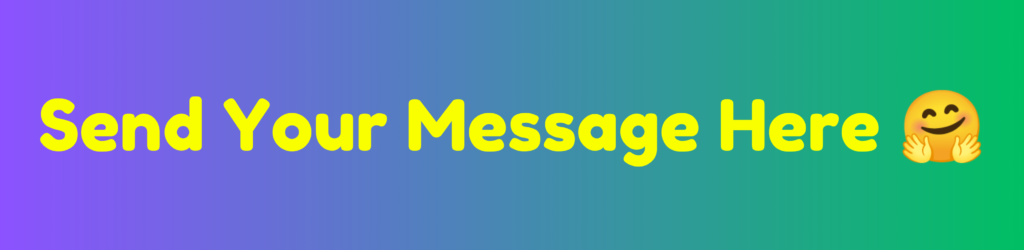
Nerve Cells and Regeneration
Nerve cells, or neurons, exhibit varying abilities to regenerate, influenced significantly by their anatomical location within the nervous system. The human nervous system is divided into two main components: the central nervous system (CNS), which comprises the brain and spinal cord, and the peripheral nervous system (PNS), including all other neural tissue throughout the body. A critical distinction lies in the regenerative capabilities of nerve cells from these two systems. PNS nerve cells have a remarkable ability to regenerate after being damaged. This regeneration process is facilitated by supportive cells, known as Schwann cells, which create a conducive environment for regrowth by producing growth factors and guiding the regrowing axons to their target areas.
In contrast, the CNS exhibits a much more limited capacity for regeneration. Following injury, neurons in the CNS often undergo a process known as Wallerian degeneration, where the damaged axon degenerates, and the surrounding environment becomes inhospitable to regeneration. Factors such as the presence of inhibitory molecules in the myelin sheath and the establishment of a glial scar contribute to this limited recovery. Consequently, CNS injuries, such as spinal cord damage, often lead to persistent functional deficits, significantly affecting the quality of life.
Recent advancements in neuroscience are focused on bridging this gap between CNS and PNS regenerative capabilities. Researchers are exploring various potential treatments, including the application of neurotrophic factors, stem cell therapies, and biomaterials to promote nerve regeneration and functional recovery in the CNS. A promising area of study involves the manipulation of the extracellular matrix to create a supportive environment for nerve cells. As understanding grows about the mechanisms that underpin regeneration, the hope is to develop effective therapies that can stimulate recovery from nerve injuries, thus enhancing the overall therapeutic outcomes for patients suffering from neurological impairments.
Frequently Asked Questions about Nerve Cells
Nerve cells, or neurons, are vital components of the nervous system, serving as the primary units responsible for transmitting information throughout the body. Below are some frequently asked questions that provide insight into their function and maintenance.
What are Nerve Cells?
Nerve cells, or neurons, are specialized cells in the nervous system that transmit electrical signals throughout the body. They play a key role in communication between the brain, spinal cord, and other body parts, enabling functions like movement, sensation, and thought.
What is the average lifespan of a nerve cell?
The lifespan of a nerve cell can vary significantly depending on its type and location in the body. Most neurons in the human brain can last a lifetime, often existing for decades. However, certain peripheral neurons, particularly those in the sensory and motor pathways, may have shorter lifespans and may regenerate throughout a person’s life.
How does stress affect nerve cells?
Stress can have significant negative effects on nerve cells. Chronic stress leads to the release of stress hormones, such as cortisol, which can impair neuronal function and contribute to the death of neurons over time. This process can affect cognitive functions and increase the risk of neurodegenerative diseases. Stress management techniques, such as mindfulness and physical exercise, can promote healthier nerve cell function.
In what ways do drugs influence nerve cells?
Various substances, particularly recreational drugs, can significantly alter the functioning of nerve cells. For instance, stimulants like cocaine increase dopamine levels, leading to heightened activity within certain neuron pathways. Conversely, depressants, such as alcohol, can slow down neuronal communication. Understanding drug effects on the nervous system is essential for mitigating potential risks and promoting overall neurological health.
What tips are there for maintaining healthy nerve cells?
Maintaining nerve cell health can be achieved through several lifestyle choices. A balanced diet rich in omega-3 fatty acids, antioxidants, and vitamins can support neuron function. Regular physical activity enhances blood flow and can stimulate the production of neurotrophic factors that promote neuron health. Additionally, mental exercises and social engagement help maintain cognitive function and encourage a healthy nervous system.
Can nerve cells regenerate after injury?
Nerve cell regeneration is a complex process. While some peripheral neurons (those outside the brain and spinal cord) can regenerate to a limited extent, neurons in the central nervous system (CNS), including those in the brain and spinal cord, have a much more limited ability to repair or regenerate after injury. Advances in medical research are exploring ways to stimulate regeneration in the CNS, but significant breakthroughs are still required.
What is the role of myelin in nerve cells?
Myelin is a fatty substance that wraps around the axons of many neurons, forming an insulating layer known as the myelin sheath. This sheath increases the speed at which electrical impulses travel along the nerve cells. Without myelin, signals would move much slower, leading to neurological disorders such as multiple sclerosis, where myelin is damaged.
Do nerve cells communicate with each other?
Yes, nerve cells communicate with each other through specialized connections called synapses. At the synapse, neurotransmitters are released from one neuron and bind to receptors on the next neuron, transmitting signals. This intricate network of communication allows the brain and nervous system to control bodily functions, respond to stimuli, and process information.
What are some common diseases or conditions that affect nerve cells?
Several conditions can impair the function or health of nerve cells, including neurodegenerative diseases like Alzheimer’s disease, Parkinson’s disease, and multiple sclerosis. Additionally, conditions like diabetic neuropathy and traumatic injuries, such as spinal cord damage, can also impact nerve function.
How does age affect nerve cells?
As we age, nerve cells tend to lose some of their regenerative abilities. There is often a gradual decline in the number of neurons, and the remaining neurons may experience decreased efficiency in transmitting signals. This decline in neural function is partly responsible for cognitive changes and the development of age-related conditions like dementia.
What is neuroplasticity?
Neuroplasticity refers to the brain’s ability to reorganize itself by forming new neural connections. This process is most active during childhood, but it continues throughout adulthood. Neuroplasticity allows the nervous system to adapt to new experiences, learn new skills, and compensate for injury. Engaging in new activities, learning, and rehabilitation exercises can help promote neuroplasticity.
How does nutrition affect nerve cell health?
Nutrition plays a critical role in the health of nerve cells. Nutrients like vitamin B12, folate, and antioxidants are essential for nerve function. Omega-3 fatty acids, found in foods like fish and flaxseeds, support the structure and function of neurons. A deficiency in these nutrients can lead to neurological problems, such as peripheral neuropathy or cognitive decline.
Are nerve cells involved in memory and learning?
Yes, nerve cells are integral to the processes of memory and learning. Neurons communicate and form complex networks that help store and retrieve information. Changes in the strength and structure of these connections, known as synaptic plasticity, are believed to be the basis of memory formation. The hippocampus, in particular, is a key area of the brain involved in memory processing.
Can nerve cells function without oxygen?
No, nerve cells require a constant supply of oxygen to function properly. Oxygen is critical for cellular respiration, which generates the energy needed for nerve cells to transmit electrical impulses. A lack of oxygen can lead to cellular damage and death, which is often observed in conditions like stroke.
What is the role of glial cells in relation to nerve cells?
Glial cells are non-neuronal cells that play supportive roles in the nervous system. They assist in maintaining the environment around neurons, providing nutrients, insulating axons, and removing waste products. Glial cells, such as oligodendrocytes and Schwann cells, also produce myelin. Additionally, microglia act as immune cells in the brain and spinal cord, responding to injury and infection.
Understanding these aspects of nerve cells can foster better awareness of one’s neurological well-being and guide healthier lifestyle choices.
Conclusion and Future Perspectives
Understanding nerve cells, or neurons, is crucial to grasping the intricate workings of the nervous system. Throughout this blog post, we have explored the fundamental structure and function of nerve cells, highlighting their essential role in communication within the body. Nerve cells not only transmit signals but also contribute to complex processes such as reflexes, perception, and cognition. The diverse types of neurons—sensory, motor, and interneurons—demonstrate the specialized functions that support overall physiological processes.
In addition to their basic functions, recent advances in neurobiology have shed light on the adaptability and plasticity of nerve cells, revealing how they can strengthen or weaken connections based on experience. This neuroplasticity has profound implications for rehabilitation following injury and the treatment of neurological disorders. As our understanding deepens, innovative therapies aimed at nerve regeneration and functional recovery are becoming increasingly viable.
Looking toward the future, there remain numerous avenues for research that hold potential for significant contributions to the field. Investigating the molecular mechanisms underlying neuron functionality could provide insights into degenerative diseases such as Alzheimer’s and Parkinson’s. Additionally, the relationship between nerve cells and other cellular components of the nervous system is an area ripe for exploration, particularly in understanding the role glial cells play in supporting neuronal health.
Moreover, technologies such as optogenetics and advanced imaging techniques offer promising tools to study nerve cell activity with unprecedented precision. Such developments may unlock further mysteries of the nervous system, paving the way for breakthroughs that enhance our understanding of brain disorders and inform targeted treatment strategies.
In conclusion, the significance of understanding nerve cells extends beyond basic science; it is imperative for the advancement of medical knowledge and therapeutic strategies. Continued research is essential to unravel the complexities of the nervous system and ultimately improve the quality of life for individuals affected by neurological conditions.

Discover more from HUMANITYUAPD
Subscribe to get the latest posts sent to your email.