Understanding Storm
A storm is a significant disturbance in the atmosphere that results in various forms of weather phenomenon. At its core, a storm typically involves changes in temperature, pressure, and moisture, which can lead to multiple types of severe weather. The characteristics of storms can vary widely, notably influencing local climates and causing impacts ranging from mild inconveniences to catastrophic events.
There are several primary types of storms, each distinguished by their unique features and forming conditions. Thunderstorms, for example, are characterized by thunder, lightning, heavy rain, and sometimes hail. These storms develop in warm, moist air, typically during the late afternoon or evening when temperatures and humidity levels peak. On the other hand, hurricanes, also known as tropical cyclones, are large, organized systems that originate over warm ocean waters. They bring strong winds, heavy rains, and can cause significant coastal flooding, making them particularly dangerous in vulnerable areas.
Tornadoes, although often associated with severe thunderstorms, are distinct in formation and behavior. These rapidly rotating columns of air can reach tremendous speeds and are capable of devastating areas within minutes. Tornadoes typically form in severe thunderstorms when conditions are right, such as the presence of warm, moist air at the surface and cooler, drier air aloft, creating an unstable atmosphere.
Blizzards, another type of storm, involve prolonged periods of heavy snowfall combined with strong winds. These storms can reduce visibility and create hazardous travel conditions, primarily occurring in regions that experience cold winter temperatures. The meteorological conditions necessary for storm formation often include the presence of low-pressure systems, warm and cold air masses, and adequate moisture in the atmosphere, collectively contributing to the dynamic and often unpredictable nature of storms.
The Formation of Storms
The formation of storms is a complex process driven by a combination of atmospheric conditions, including instability, moisture, and temperature gradients. At the heart of storm development lies the concept of atmospheric instability. This occurs when warm, moist air near the surface rises, displacing cooler air above it. As this warm air ascends, it cools and condenses, forming clouds. The rising motion of the air can lead to the creation of thunderclouds, or cumulonimbus clouds, which are often associated with thunderstorms and severe weather.
Moisture is another critical component in the formation of storms. The presence of humidity in the air provides the necessary water vapor that condenses into droplets, leading to cloud formation. When sufficient moisture accumulates in the atmosphere, particularly in conjunction with temperature variations, this can result in the development of various storm types. For instance, tropical storms and cyclones typically form over warm ocean waters, where the heat adds additional energy to the moisture-laden air, intensifying the storm.
Temperature gradients contribute significantly to storm formation as well. When there is a notable difference between the temperatures of different air masses—such as a warm, moist air mass clashing with a cold, dry one—the resulting instability can trigger the initiation of storms. This interaction is crucial in the development of frontal storms, which occur along the boundaries between different air masses. In these scenarios, the warm air is forced to rise over the colder air, leading to cloud formation and potential precipitation.
Understanding these fundamental processes provides insight into why storms can vary widely in strength and type. From thunderstorms that roll across the plains to the devastating power of tropical cyclones, each storm is a testament to the intricate workings of our atmosphere.
The Anatomy of a Thunderstorm
Thunderstorms are complex meteorological systems characterized by significant atmospheric instability and are essential in the Earth’s climate system. Their structure consists primarily of three main components: the updraft, the downdraft, and the anvil-shaped cloud formation known as the cumulonimbus cloud. Understanding these elements can provide insight into the behavior and lifecycle of thunderstorms.
The updraft is a crucial component of a thunderstorm, as it represents the warm, moist air that rises rapidly. This rising air cools adiabatically, leading to condensation and the formation of water droplets. This process not only generates clouds but also releases latent heat, which further fuels the storm. As the updraft strengthens, it can reach heights of over 10 kilometers, forming the towering structure typical of severe thunderstorms.
In contrast, the downdraft occurs when the cooled air becomes denser and begins to descend. This sinking air often brings with it precipitation, such as rain or hail, which can be quite severe. The interaction between the updrafts and downdrafts is essential for the storm’s maintenance and development; the turbulence created leads to various phenomena associated with thunderstorms, including lightning and flash flooding.
As thunderstorms evolve, they undergo distinct lifecycle stages: the cumulus stage characterized by the initial growth of the cloud, the mature stage where both updrafts and downdrafts are present, and finally, the dissipating stage when the downdrafts dominate, leading to a cessation of storm activity. Lightning, a notable feature of thunderstorms, occurs due to electrical discharges in the atmosphere, while hail forms when strong updrafts carry water droplets into freezing regions of the storm. Collectively, these elements illustrate the dynamic forces at play in thunderstorms, showcasing nature’s formidable power.
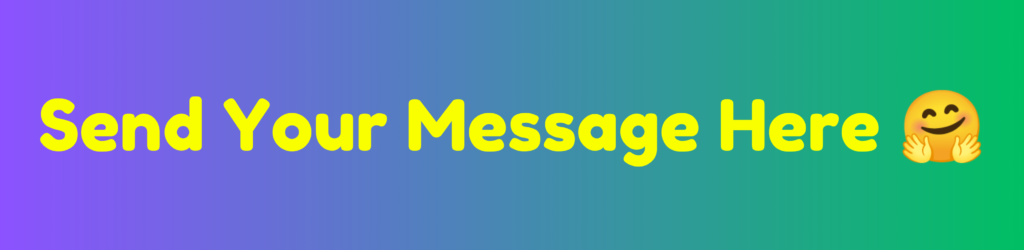
Understanding Hurricanes and Typhoons
Hurricanes and typhoons represent some of the most powerful natural phenomena on Earth, classified as tropical storms that arise over warm ocean waters. These storms form during specific conditions, typically when sea surface temperatures reach 26.5 degrees Celsius (about 80 degrees Fahrenheit) or higher, allowing for the evaporation of vast amounts of water, which fuels convection currents in the atmosphere. The rotation of the Earth further contributes to the development of these formidable weather systems through the Coriolis effect, which encourages their circular motion.
Once a tropical storm reaches sustained winds of 74 miles per hour (119 kilometers per hour), it is categorized as either a hurricane or a typhoon, depending on its location. In the North Atlantic and the Northeast Pacific, these storms are referred to as hurricanes, while those that form in the Northwest Pacific are called typhoons. The Saffir-Simpson Hurricane Wind Scale is a crucial tool that classifies hurricanes into five distinct categories based on their wind speeds and potential damage. Category 1 storms are the least severe, causing minimal damage, while Category 5 storms can lead to catastrophic destruction, with winds exceeding 157 miles per hour (252 kilometers per hour).
The dangers posed by these powerful storms extend beyond strong winds. Storm surges, which involve the rapid rise in sea levels due to the intense low pressure and strong winds, can inundate coastal areas and lead to extensive flooding. Additionally, hurricanes and typhoons bring heavy rainfall, which can result in inland flooding and landslides. Understanding the formation and classification of these storms is essential for preparedness and mitigation efforts, helping individuals and communities better respond to the threats posed by hurricanes and typhoons.
Tornado Formation and Structure
Tornadoes represent one of nature’s most violent phenomena, characterized by rapidly rotating columns of air. These formidable storms typically develop from severe thunderstorms, particularly supercells, which are a type of rotating thunderstorm that possesses a defined rotating updraft known as a mesocyclone. Tornado formation involves a complex series of atmospheric conditions, primarily requiring a combination of warm, moist air from the surface, cold, dry air aloft, and strong wind shear—the variation of wind speed and direction with height.
The development of a tornado begins with the formation of a supercell. As the warm air rises, it cools and condenses, forming a cumulonimbus cloud. When wind shear is strong enough, the rotating updraft can organize and develop into a mesocyclone. If this mesocyclone becomes tight and extends downward, it may generate a visible funnel cloud. A tornado is officially classified when the funnel cloud makes contact with the ground. The most intense tornadoes are often associated with an enhanced Fujita (EF) scale rating of EF3 or higher, which indicates wind speeds exceeding 136 miles per hour.
Statistically, tornadoes occur most frequently in the United States, particularly within an area known as “Tornado Alley,” which includes states like Texas, Oklahoma, and Kansas. On average, the U.S. experiences approximately 1,000 tornadoes each year, with varying intensity and duration. Most tornadoes are relatively weak and short-lived, but a small percentage are classified as strong or violent, capable of causing extensive damage and loss of life. Understanding the formation and characteristics of tornadoes is crucial not only for improving prediction and warning systems but also for enhancing community preparedness and response strategies in areas prone to these devastating storms.
The Role of Climate Change in Storm Intensity
Climate change is increasingly recognized as a pivotal factor impacting the intensity and frequency of storms globally. Scientific research suggests that as the Earth’s average temperature rises due to the accumulation of greenhouse gases in the atmosphere, various atmospheric and oceanic processes are altered. This shift has significant implications for storm formation and behavior.
Rising sea surface temperatures are a key component in this dynamic. Warmer waters provide more heat and moisture, essential fuel for storm systems. Studies indicate a correlation between elevated ocean temperatures and the increased rapid intensification of storms, particularly hurricanes. For instance, during the last few decades, the Atlantic hurricane season has seen a marked increase in the number of storms reaching major hurricane status, largely attributed to warmer ocean temperatures.
Moreover, shifting weather patterns associated with climate change contribute to the evolution of storm frequency. Changes in atmospheric circulation can create conditions conducive to more severe weather events, including increased likelihoods of extreme rainfall and drought, which in turn can lead to flooding and other storm-related impacts. The relationship between climate change and atmospheric circulation patterns, such as the jet stream, remains a critical area of research.
Additionally, extreme weather events tend to become more prevalent as climate change progresses. Research highlights that not only are storms becoming more intense, but their impacts are also affecting broader regions. As communities adapt to rising sea levels and increased storm surge risks, understanding the connections between climate change and storm patterns will be vital for disaster preparedness and mitigation strategies.
To summarize, the intricate connection between climate change and storm intensity merits further scientific inquiry. By enhancing our comprehension of these relationships, we can better prepare for the challenges posed by an increasingly volatile climate, ensuring that communities globally can mitigate the risks associated with more severe storm events.
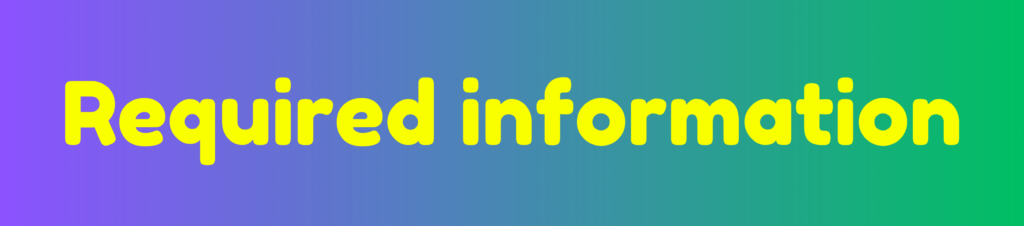
Storm Prediction and Preparedness
Storm prediction plays a crucial role in mitigating the impact of severe weather on communities. Meteorologists utilize advanced technologies such as radar, satellite data, and computer modeling to forecast the development and path of storms. Radar systems provide real-time data on precipitation, wind patterns, and storm movement, enabling scientists to identify areas at risk. Satellite images allow for a comprehensive view of weather systems, helping to monitor the evolution of storms from their inception to landfall.
Computer modeling is integral to the prediction process. These models simulate the atmosphere’s behavior based on current conditions and historical data, aiding in forecasting storm intensity, rainfall, and potential hazards. The accuracy of these predictions can significantly affect public safety, enabling individuals and infrastructure to prepare for severe weather events. The National Weather Service and other meteorological agencies often issue warnings based on these predictive models, alerting the public to take necessary precautions.
Preparedness is equally important in the face of impending storms. Individuals and communities are encouraged to develop emergency plans well in advance of storm season. This involves understanding local evacuation routes, establishing communication plans, and creating comprehensive emergency kits that include essential supplies such as food, water, first-aid items, and flashlights. Awareness of safety protocols, such as seeking shelter during a storm or avoiding floodwaters, is critical for minimizing injuries and fatalities during severe weather events.
Educational programs aimed at raising awareness about storm preparedness can significantly enhance community resilience. By fostering a culture of preparedness, communities can better withstand the impact of storms, thereby reducing the risks associated with nature’s fury. Engaging in discussions about storm prediction and preparedness not only equips individuals with necessary knowledge but also builds a stronger, more informed society ready to face such challenges together.
The Impact of Storms on the Environment and Society
Storms represent a significant challenge to both natural ecosystems and human communities. The physical and environmental impacts of storms can be profound, often leading to flooding, soil erosion, and habitat destruction. Flooding, a common consequence of heavy rainfall associated with storms, can submerge ecosystems, disrupt aquatic habitats, and significantly alter landscapes. This not only threatens the survival of various flora and fauna but can also lead to long-term changes in the ecological balance of affected areas.
Soil erosion is another critical effect triggered by powerful storms. Intense winds and heavy rains can wash away topsoil, diminishing soil fertility and jeopardizing agricultural productivity. This loss of productive land can push local communities into food insecurity, making them increasingly vulnerable to economic hardship. Furthermore, the reduction of soil quality increases the risk of landslides, fundamentally altering land management and agricultural strategies in storm-prone regions.
The socio-economic impacts of storm-related disasters can be devastating. Infrastructure, such as roads and bridges, often suffers significant damage, leading to disruption in transportation and access to essential services. This disruption can result in economic losses and increased costs for recovery and rebuilding. Communities may experience a decline in property values, affecting local economies and diminishing the overall quality of life. Moreover, the psychological impact of such disasters on individuals and families, many of whom may lose their homes or livelihoods, cannot be overstated.
Adapting to the challenges posed by storms is crucial for mitigating their impacts on society and the environment. Communities must develop and implement effective disaster preparedness plans, invest in resilient infrastructure, and engage in sustainable land-use practices. Such measures can help reduce vulnerability to storms and protect both ecosystems and populations in the face of nature’s fury.
FAQs: Understanding Storm
Storms are complex natural phenomena that evoke curiosity and concern. Below are some frequently asked questions that delve into various aspects of storms, aiming to enhance understanding and safety.
What are the different types of storms?
Storms can be categorized into several types, including thunderstorms, hurricanes, tornadoes, blizzards, and cyclones. Each type has distinct characteristics, formation processes, and impacts on the environment. For instance, thunderstorms often produce heavy rain and lightning, while hurricanes are large, organized systems characterized by intense winds and heavy rain over ocean waters.
How can I stay safe during a storm?
Safety during storms is paramount. For thunderstorms and tornadoes, seek shelter in interior rooms, basements, or sturdy structures. When facing hurricanes or floods, it is essential to have an emergency kit ready, stay informed through weather updates, and evacuate if instructed by authorities. Knowing your area’s storm history can help inform preparedness measures.
What historical storms should I know about?
Understanding historical storms can provide insight into the severity and impact of these events. Notable storms such as Hurricane Katrina in 2005 and the Great Galveston Hurricane of 1900 serve as crucial reminders of the importance of preparedness and response measures. Analyzing the data from these storms can help improve future forecasting and response strategies.
What are less common storm types?
In addition to the familiar storms, other less common phenomena include derechos, waterspouts, and microbursts. Derechos are widespread, long-lived wind storms associated with thunderstorms, while waterspouts are tornadoes that form over water. Microbursts are sudden, powerful downdrafts that can cause extensive damage over relatively small areas.
By familiarizing oneself with these aspects of storms, individuals can gain a greater appreciation for nature’s fury and improve safety measures against unpredictable weather events.
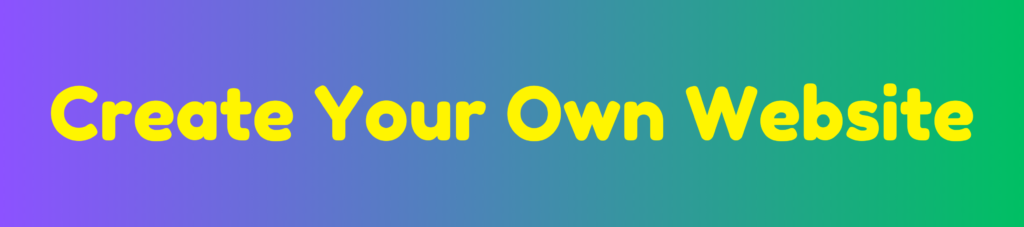
Discover more from HUMANITYUAPD
Subscribe to get the latest posts sent to your email.